The main research topics of the Stockner Lab include membrane transporters from the primary active ABC transporter family and the secondary active neurotransmitter transporters as well as TRP ion channels. The main goal is to achieve a comprehensive understanding of protein function as well as the influence by membrane environment. Molecular Dynamics (MD) simulations can in principle provide insights with unrivalled time and positional resolutions, but require experimental data to provide verification and to set boundary conditions. We use modelling and simulation techniques as the platform for integrating experiments and theory. Experiments using pharmacological and electrophysiological approaches are conducted within the Stockner Lab, and frequently with collaboration partners.
Key to the success of research on the complex molecular machines is interdisciplinarity through combining experiments with modelling and simulations, because no method alone can answer the complex questions that link structure with protein dynamics, function, binding an the driving forces energising structural changes and thus function. Our main areas of research include:
- Structure, function and dynamics of transporters and channels
- The transport cyle or secondary active transporters
- Gating and ion permeation of TRP ion channels
- Protein lipid interactions and functional roles of lipids
- Interactions of substrate and inhibitors with transporters
- Enthalpy, entropy and kinetics
- Energy coupling of transporters
The human genome codes for 48 members of the ABC protein family. Most members are membrane transporters, while four are involved in regulation of gene expression. The membrane proteins assemble to 42 functional membrane transporters. Common to all ABC transporters are the nucleotide binding domains, which provide the driving force through ATP hydrolysis. The transmembrane domains form the substrate recognition and translocation path, can be of several folds, and the sequence identity is also very low among subfamily members. We focus on conformational changes of the transport cycle, substrate selectivity, and coupling between ATP hydrolysis and substrate transport.
Research examples: The primary active transporter from the ABC family are essential for cell function by carrying out substrate transport. We are using free energy calculations to dissect forces and energy profile leading to energy conversion from the chemical energy of ATP hydrolysis to mechanical energy (Biophy J 2018; Sci Rep 2020), created and experimentally verified the first ABCB1 variant that is active despite degeneration of one of the nucleotide binding site (PLOS Genet. 2020), to dissect key steps associated with the transport cycle (Mol Pharm 2017; Nat Comm. 2019; Febs L 2020) and transporter asymmetry (Febs L 2020).
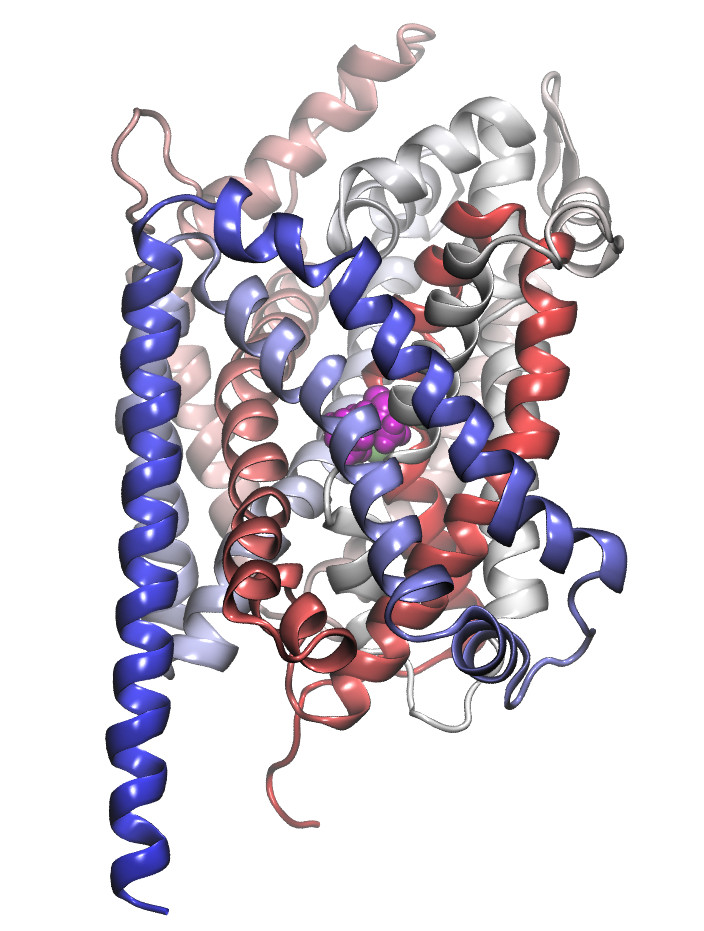
The secondary active transporters (SoLute Carriers or SLC) are a very large superfamily of membrane proteins. The human genome including over 450 members. The can be symporters, antiporters, exchangers or unidirectional transporters, and substrate translocation can be coupled or uncoupled to co-transported ions. We focus on neuronal transporters of the SLC6 family, mainly on the transporters for dopamine (DAT), norepinephrine (NET), serotonin (SERT), GABA (GAT1, 2, 3, BGT1) and creatine (CT1). Their core physiological role is termination of neurotransmission by rapid removal of neurotransmitters from the synaptic cleft, while the creatine transporter is essential for energy provision to neurons. Transporter disregulation is associated with disorders such as depression, attention deficit hyperactivity disorder, schizophrenia, seizures, autism or bipolar disorder. We are investigating substrate and inhibitor binding, and the molecular details of active transport and use experimental approaches to very predictions to eventually obtain a comprehensive understanding of transport function at the molecular level.
Research examples: At a synapse, the physiological role of secondary active transporters of the SLC6 family is to terminate neurotransmission by rapid neurotransmitter removal. We combine in-silico and in-vitro approaches to investigate transporter structures, conformations, substrate and inhibitor binding and the transport cycle (JBC 2022; JBC 2019; PNAS 2019; Angew. Chem 2016). Computations methods range from structure prediction using homology modelling, docking to MD simulations and free energy calculations using enhanced sampling methods (PLOS Comput Biol 2016; PLOS Comput Biol 2018; PLOS Comput Biol 2013, Mol Pharm 2016).
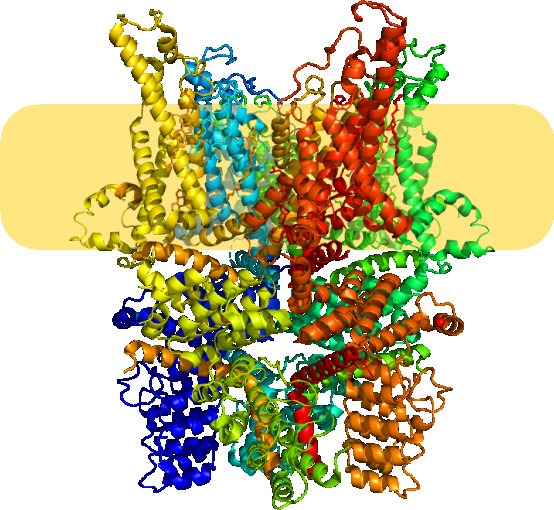
Transient Receptor Potential (TRP) channels are ligand gated Ca2+ channels, but in absence of calcium, also conduct other ions such as Na+ or K+. These voltage independent channel were original identification as a key players of visual transduction, but have now emerged as essential elements, being involved in many Ca2+ dependent physiological roles throughout the body. The successful structure determination by single particle cryo-EM experiments was an important recent breakthrough that now allows to carry out structure based approaches. We are using computational approaches including molecular modelling, MD simulations, and free energy calculations to study ligand interactions with TRP channels, channel function, dynamics, gating and ligand interaction. This interdisciplinary research project combines computational approaches with experiments, carried out by collaboration partners. Approaches include molecular biology and biophysical approach to modulate channel function by side directed mutagenesis and pharmacology, channel expression and localisation, confocal microscopy, electrophysiology and photopharmacology. The key aim is to gain a full understanding of the TRP channel gating machinery and its functional regulation.
TRP channels are seen as novel pharmacological targets, because involved in calcium signalling and thus cellular regulation. The available structures, together with our theoretical and experimental approaches are expected to serve for a rational development of ligands.
Research examples: Structural information is essential to understand channel function. Creating models of TRPC channels before the appearance of cryo-EM structures allowed for rational mutagenesis studies to probe into channel function (PNAS 2011; Cell Calcium 2013; Nat Chem Biol 2018). Combining MD simulation with mutagenesis and functional studies, we could identify the binding site of regulatory lipids (EMBO Reports 2022), the interaction of annular lipid (Biomolecules 2022) and channel activation of photo-sensitive lipids (Biomolecules 2022)
Cell membranes are a thin, flexible diffusion barrier that encloses all cell interior component of living cells. Transporters allow for substrate permeation, which is essential for sustaining cellular life. Membrane proteins constitute approximately 50% of the cell membrane surface including transporters, receptors, enzymes, channels and adhesion molecules. Substrate transport across cell membranes ensures uptake of nutrients and the removal of waste products. Cell membranes are bilayers consisting of phospholipids, sphingolipids and cholesterol and form a double layer, whereby the hydrophilic heads are exposed to the membrane surround water, while the hydrophobic tails oriented towards the membrane interior. These bilayers are impermeable for water, ions and hydrophilic compounds.
Membrane proteins are regulated in a complex way by the membrane. The biophysical properties of the membrane, such as thickness, stiffness, fluidity, bending properties exert a general impact on membrane function. These properties are dictated by the membrane composition, which can vary strongly between biological membranes. Direct protein-lipid interactions can regulate protein function, for example the signalling diacyl-glycerol (DAG) regulates TRP channels and monoamine transporters via direct interactions to the L2 lipid binding site (EMBO Reports 2022). Essential for this process are the lipids in the first lipid shell surrounding membrane proteins. These are divided into annular lipid, which shows rapid diffusion in and out of the first lipidation shell, and non-annular lipids, which remain firmly bound to proteins and are structural lipids that stabilize protein structures or are signalling lipids that tune protein functions.
Research examples: The membrane environment plays a crucial role in the regulation of transporter structure, dynamics and activity. We combined experiments with modelling and MD simulations to investigated the oligomeric states of the dopamine transporter (PLOS Comput Biol, 2018; JBC 2019), the serotonin transporter (PNAS 2013; Nat Comm 2017) and the role of PIP2. For LeuT we showed how the environment modulates the conformational equilibrium of LeuT (PLOS Comput Biol 2016; NeuroChem Res. 2020). A quantification of the effects of an anesthetic drug on the membrane pressure and its impact on protein structures (JACS 2010) showed that drugs could in principle trigger conformational changes in proteins.